Water, like any chemical substance, can exist in a gaseous, liquid or solid state… but that’s not all! When sufficiently heated and pressurized, it becomes a supercritical fluid, halfway between a liquid and a gas. Jacques Fages, a researcher in process engineering, biochemistry and biotechnology at IMT Mines Albi, answers our questions on these fluids which, among other things, can be used to replace polluting industrial solvents or dispose of toxic waste.
What is a supercritical fluid?
Jacques Fages: A supercritical fluid is a chemical compound maintained above its critical point, which is defined by a specific temperature and pressure. The critical pressure of water, for example, is the pressure beyond which it can be heated to over 100°C without becoming a gas. Similarly, the critical temperature of CO2 is the temperature beyond which it can be pressurized without liquefying. When the critical temperature and pressure of a substance are exceeded at the same time, it enters the supercritical state. Unable to liquefy completely under the effect of temperature, but also unable to gasify completely under the effect of pressure, the substance is maintained in a physical state between a liquid and a solid: its density will be equivalent to that of a liquid, but its fluidity will be that of a gas.
For CO2, which is the most commonly used fluid in supercritical state, the critical temperature and pressure are relatively low: 31°C and 74 bars, or 73 times atmospheric pressure. Because CO2 is also an inert molecule, inexpensive, natural and non-toxic, it is used in 90% of applications. The critical point of water is much higher: 374°C and 221 bars respectively. Other molecules such as hydrocarbons can also be used, but their applications remain much more marginal due to risks of explosion and pollution.
What are the properties of supercritical CO2 and the resulting applications?
JF: Supercritical CO2 is a very good solvent because its density is similar to that of a liquid, but it has much greater fluidity – similar to that of a gas – which allows it to penetrate the micropores of a material. The supercritical fluid can selectively extract molecules, it can also be used for particle design.
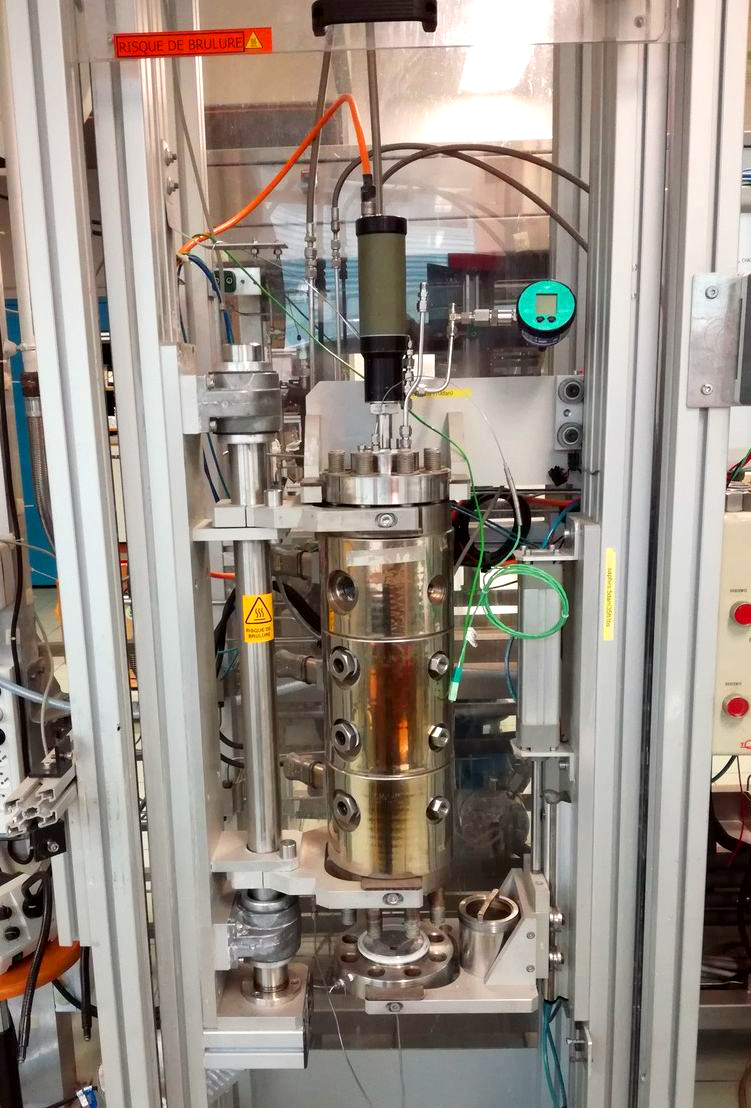
A device designed for implementing extraction and micronization processes of powders.
Supercritical CO2 can be used to clean medical devices, such as prostheses, in addition to the sterilization methods used. It removes all the impurities to obtain a product that is clean enough to be implanted in the human body. It is a very useful complement to current methods of sterilization. In pharmacy, it allows us to improve the bioavailability of certain active principles by improving their solubility or speed of dissolution. At IMT Mines Albi, we worked on this type of process for Pierre Fabre laboratories, allowing the company to develop its own research center on supercritical fluids.
Supercritical CO2 has applications in many sectors such as materials, construction, biomedical healthcare, pharmacy and agri-food as well as the industry of flavorings, fragrances and essential oils. It can extract chemical compounds without the use of solvents, guaranteeing a high level of purity.
Can supercritical CO2 be used to replace the use of polluting solvents?
JF: Yes, supercritical CO2 can replace existing and often polluting organic solvents in many fields of application and prevents the release of harmful products into the environment. For example, manufacturers currently use large quantities of water for dyeing textiles, which must be retreated after use because it has been polluted by pigments. Dyeing processes using supercritical CO2 allow textiles to be dyed without the release of chemicals. Rolls of fabric are placed in an autoclave, a sort of large pressure cooker designed to withstand high pressures, which pressurizes and heats the CO2 to its critical state. Once dissolved in the supercritical fluid, the pigment permeates to the core of the rolls of fabric, even those measuring two meters in diameter! The CO2 is then restored to normal atmospheric pressure and the dye is deposited on the fabric while the pure gas returns into the atmosphere or, better still, is recycled for another process.
But, watch out! We are often criticized for releasing CO2 into the atmosphere and thus contributing to global warming. This is not true: we use CO2 that has already been generated by an industry. We therefore don’t actually produce any and don’t increase the amount of CO2 in the atmosphere.
Does supercritical water also have specific characteristics?
JF: Supercritical water can be used for destroying hazardous, toxic or corrosive waste in several industries. Supercritical H2O is a very powerful oxidizing environment in which organic molecules are rapidly degraded. This fluid is also used in biorefinery: it gasifies or liquefies plant residues, sawdust or cereal straw to transform them into liquid biofuel, or methane and hydrogen gases which can be used to generate power. These solutions are still in the research stage, but have potential large-scale applications in the power industry.
Are supercritical fluids used on an industrial scale?
JF: Supercritical CO2 is not an oddity found only in laboratories! It has become an industrial process used in many fields. A French company called Diam Bouchage, for example, uses supercritical CO2 to extract trichloroanisole, the molecule responsible for cork taint in wine. It is a real commercial success!
Nevertheless, this remains a relatively young field of research that only developed in the 1990s. The scope for progress in the area remains vast! The editorial committee of the Journal of Supercritical Fluids, of which I am a member, sees the development of new applications every year.