Temporary tattoos for brain exploration
A team of bioelectronics researchers at Mines Saint-Étienne has developed a new type of electroencephalogram electrode using a temporary tattoo technique. As effective as traditional electrodes, but much more comfortable, they can provide extended recordings of brain activity over several days.
The famous decalcomania transfer technique – made popular in France by the Malabar chewing gum brand in the 1970s – has recently come back into fashion with temporary tattoos. But it does not serve solely to provide fun for people all ages. A new use has been developed with the invention of temporary tattoo electrodes (TTE) designed to record electrophysiological signals.
Originally developed to pick up heart (electrocardiogram, ECG) and muscle signals (electromyogram, EMG), the technique has been refined to reach the holy Grail of bioelectronics: the brain. “Electroencephalographic signals (EEG) are the hardest to record since their amplitudes are lower and there is more background noise, so it was a real challenge for us to create flexible epidermal electronic devices that are as effective as standard electrodes,” explains Esma Ismailova a bioelectronics researcher at Mines Saint-Étienne.
From Pontedera to Saint-Étienne
The process for printing tattoo electrodes was developed by an Italian team, led by Francisco Greco, at the Italian Institute of Technology in Pontedera. The next step for preclinical application was carried out at the Saint-Etienne laboratory. Laura Ferrari, a PhD student who worked on TTE with Francisco Greco for her thesis, chose to carry out postdoctoral research with Esma Ismailova in light of her experience in the field of wearable connected electronics. In 2015, the Mines Saint-Étienne team had developed a connected textile, derived from the technique used to print on kimonos, intended to record an electrocardiogram on a moving person, with fewer artifacts than traditional ECG electrodes.
The sensors of the tattoo electrodes, like the textile electrodes, are composed of semi-conductive polymers. These organic compounds, which were the topic of the 2000 Nobel prize in chemistry, act as transistors and offer new possibilities in the field of surface electronics. The conductive polymer used is called PEDOT:PSS. It is mixed with ink and projected on a paper sold commercially for temporary tattoos, using a regular inkjet printer. The back layer is removed at the time of application. A simple wet sponge dissolves the soluble layer composed of cellulose, and the tattoo is transferred to the skin. The materials and techniques used in the microfabrication process for TTEs make it suitable for large-scale, low-cost production.
Esma Ismailova and her team worked extensively on the assembly and interconnection between the electrodes and electronic signal recording devices. An extension ending in a plastic clip was manufactured through 3D printing and integrated in the decalcomania. The clip makes it possible to attach a wire to the tattoo: “We had to solve the problem of transmitting the signal to transfer the data. Our goal is now to develop embedded electronics along with the electrodes, a microfabricated, laminated board on the patch to collect and memorize information, or transmit it through a mobile phone,” says the Saint- Étienne researcher.
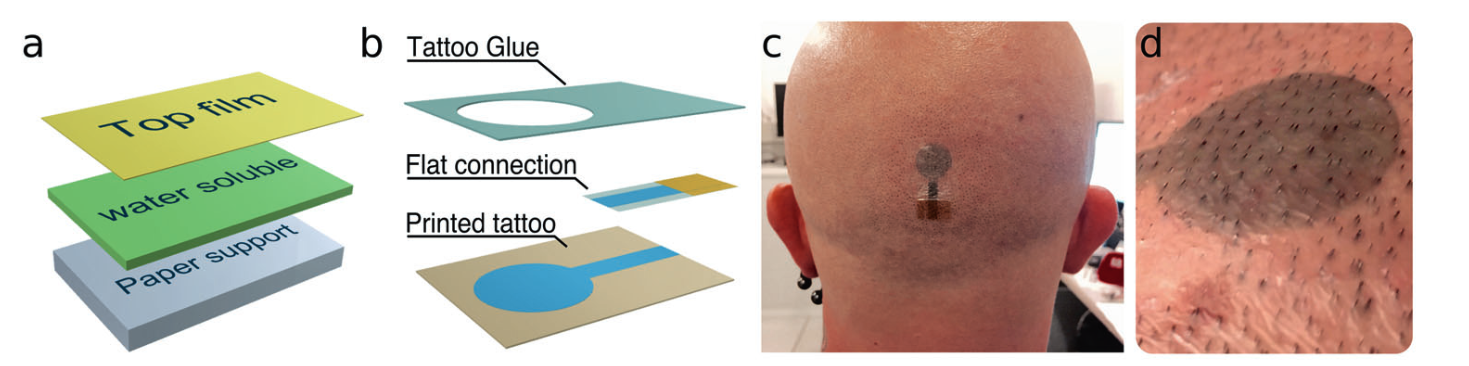
a: multi-layer structure of a TTE allowing for the transfer of the top film on which the electrode is printed b: exploded view of a TTE with integrated flat connection c: TTE transferred to the scalp in the occipital region d: close-up of a TTE 12h after application with hair regrowth
Electrodes that are more comfortable for patients…
The dry electrodes composed of a one-micron thick polymer film conform perfectly to the surface of the skin due to their flexibility. This interface makes it possible to dispense with the gel which is necessary for traditional electrodes, which dries out after a few hours, making the electrodes inoperative. The transfer must be done on shaved skin, but a study has shown that hair regrowth through the film does not stop them from being effective. This means that they can be used for 2 to 3 days, provided they do not get wet, since the principle of temporary tattoos is that they are broken down by washing with soap and water. Research is currently underway to replace the regular transfer layer by a more resistant, water-repellent material, which would extend their lifetimes.
For Esma Ismailova, this technology is a huge step forward, for both the field of clinical research and patient care: “These new flexible, stretchable, very thin electrodes are ergonomic, conformable, virtually imperceptible, and are therefore much more acceptable for patients, particularly children and elderly people, for whom certain exams can be stressful.” Indeed, to perform an EEG, patients must normally wear a headset that attaches below the chin, composed of electrodes on which the technician applies gel.
… and more effective for doctors
Another advantage of these temporary tattoo electrodes is their compatibility with magnetoencephalography (MEG). Since they are composed entirely of organic materials and therefore do not contain any metal, they do not disturb the magnetic field generated by the device and do not create artifacts, so they can be used to perform EEGs coupled with MEGs. These two techniques for exploring neuronal activity are complementary and refine information about the starting point of epileptic seizures, the review of systems for certain tumors before their ablation, and neurodegenerative diseases.
The clinical assessment of TTE in the field of neurophysiology was carried out in collaboration with Jean-Michel Badier from the Institut de Neurosciences des Systèmes at the University of Aix-Marseille. This study was recently published in the journal Nature, and confirmed that their performance was similar to traditional electrodes for standard EEG, and superior for MEG, since they do not produce any shadow areas.
“We’ve done a proof of concept, now we’re trying to develop a device that can be used at home. We plan to do a study with epileptic or autistic children, for whom comfort and acceptability are very important,” explains Esma Ismailova. These tattoo electrodes – like other connected technology –will generate a great amount of data. For the researcher, “it’s essential to collaborate with researchers who can process this data using specialized algorithms. It’s a new era for smart wearables designed for personalized, preventive medicine, in particular through the early detection of abnormalities.”
Sarah Balfagon